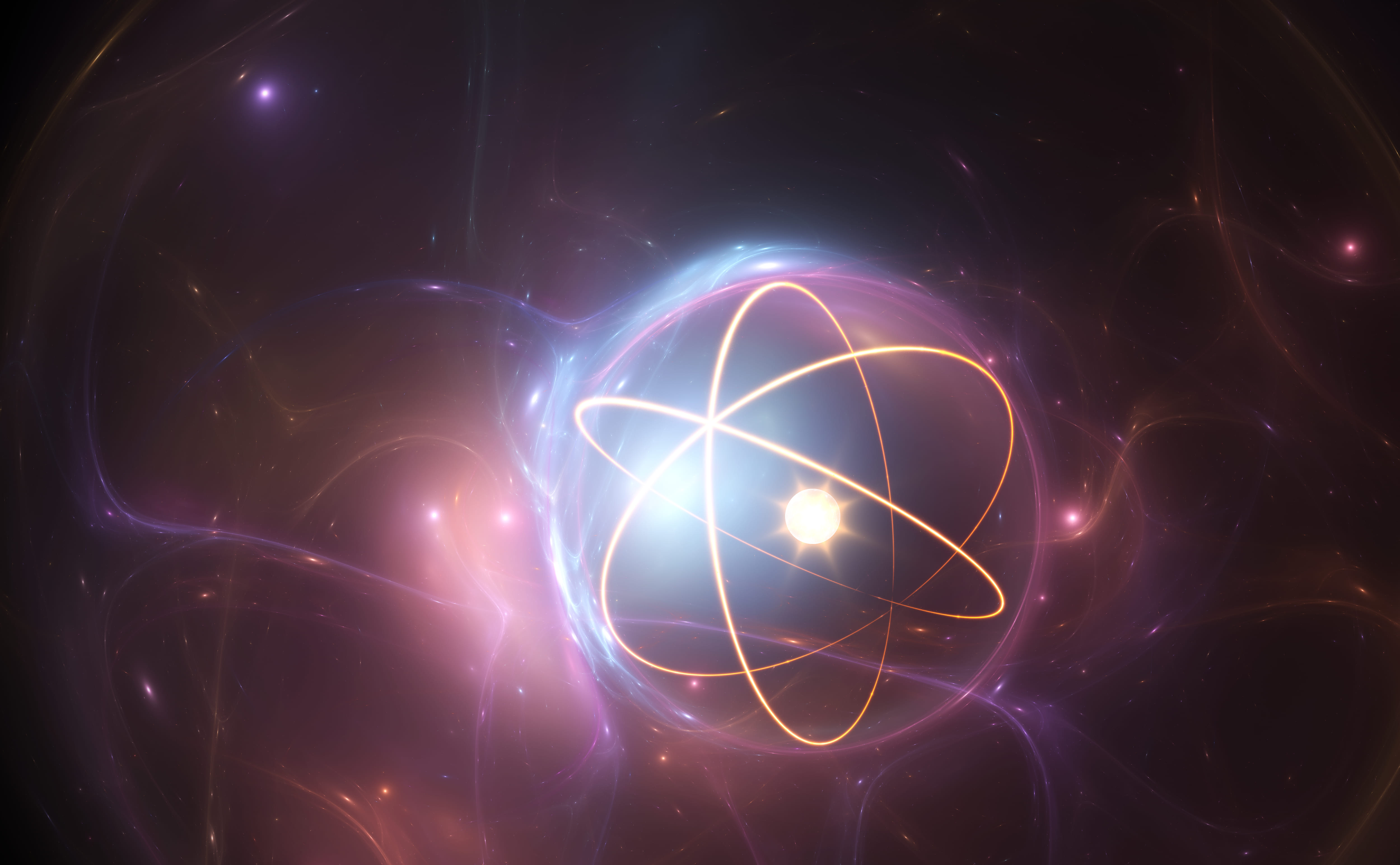
Quantum information processing with photonic and atomic qubits
The interaction between photonic and matter qubits represents an exciting and promising avenue for the development of quantum computers. Quantum computing harnesses the principles of quantum mechanics to process information in ways beyond the capacity of classical computers, offering the potential for exponential speedup in solving specific problems. Error correction poses a critical challenge in quantum computing because quantum bits(qubits) are inherently susceptible to environmental noise and other sources of interference. The interaction of photonic-matter qubit plays a crucial role in this endeavor, offering an advantage over “single species” qubits by harnessing the best of both worlds.
Photonic qubits are much less restricted by spatial considerations, enabling a remarkable level of long distance and non-trivial connectivity between qubits. However, photons have a short lifespan, primarily suiting them for measurement-based quantum computation. Yet, high loss rates due to interaction with practically anything will likely persist as a problem for photonic qubits in the foreseeable future.
Matter-based qubits bring stability to the table, manifested in long coherence times that make them good candidates for quantum memories. Combining the two types of qubits in a dual architecture that allows for both high connectivity, and significant scalability, opens the door for the experimental realization of new error-correcting codes such as Quantum Low Density Parity Check codes, which are waiting to revolutionize the world of quantum computing.
Atomic qubits exemplify matter-based qubits that interact significantly well with photons, enabling the aforementioned dual architecture. To generate quantum error correction codes within such an architecture, one requires the capability to prepare atomic and photonic qubits, manipulate them using single- and two-qubit gates (including entangling gates), and measure the qubits' states.
Mature technological solutions already exist for preparing and number-resolving measurements of photonic qubits via linear optics devices and SNSPD detectors. However, the preparation and measurement of atomic states are more complex, often involving external electromagnetic fields in the form of slow and intricate active control pulses.
A more efficient approach involves utilizing a swap gate between atomic and photonic qubits. This allows for the preparation of the atom to any state on the Bloch sphere and the transfer of this state to a photonic qubit, all within the timescale of a single photon's interaction with the atom - typically a few nanoseconds.
Detailed analysis of the SWAP interaction
The work published in [1] explores the manifestation of a swap gate within a Cavity Quantum Electrodynamics (CQED) system. These physical systems leverage the interaction between atoms and photons confined in a cavity. Here, a cavity refers to an optical resonator capable of trapping and enhancing the interaction between light and matter.
This study investigates a system comprising a three-level atom in a configuration coupled to a ring-shaped resonator. The underlying process, termed as Single Photon Raman Interaction (SPRINT), is utilized to deterministically execute a set of gates between a single photon and an atom. The analysis primarily concentrates on the swap gate, which was demonstrated experimentally shortly afterwards [2], and conducts an extensive theoretical analysis, supported by numerical simulations. This analysis aims to characterize the gate’s performance and identify optimal system parameters considering realistic loss and error mechanisms.
This work shows that these loss and error mechanisms can be suppressed by a smart choice of tunable system parameters, leading to a cavity-QED photon-atom swap gate that can be performed with high fidelity, and can be scaled up to enable fault-tolerant quantum computing. Moreover, the fidelity and loss can be further improved through better engineering of the photonic ring resonators, a vibrant field of study in both academia and industry. Incorporating such a robust cavity-QED swap gate along with an entangling photon-atom qubit gate, and the aforementioned linear optics and detector technologies, completes the set of tools needed for creating a scalable and fault-tolerant quantum computing technology.
[1] S. Rosenblum, A. Borne and B. Dayan, Physical Review A. 95, 033814 (2017)
categories
popular
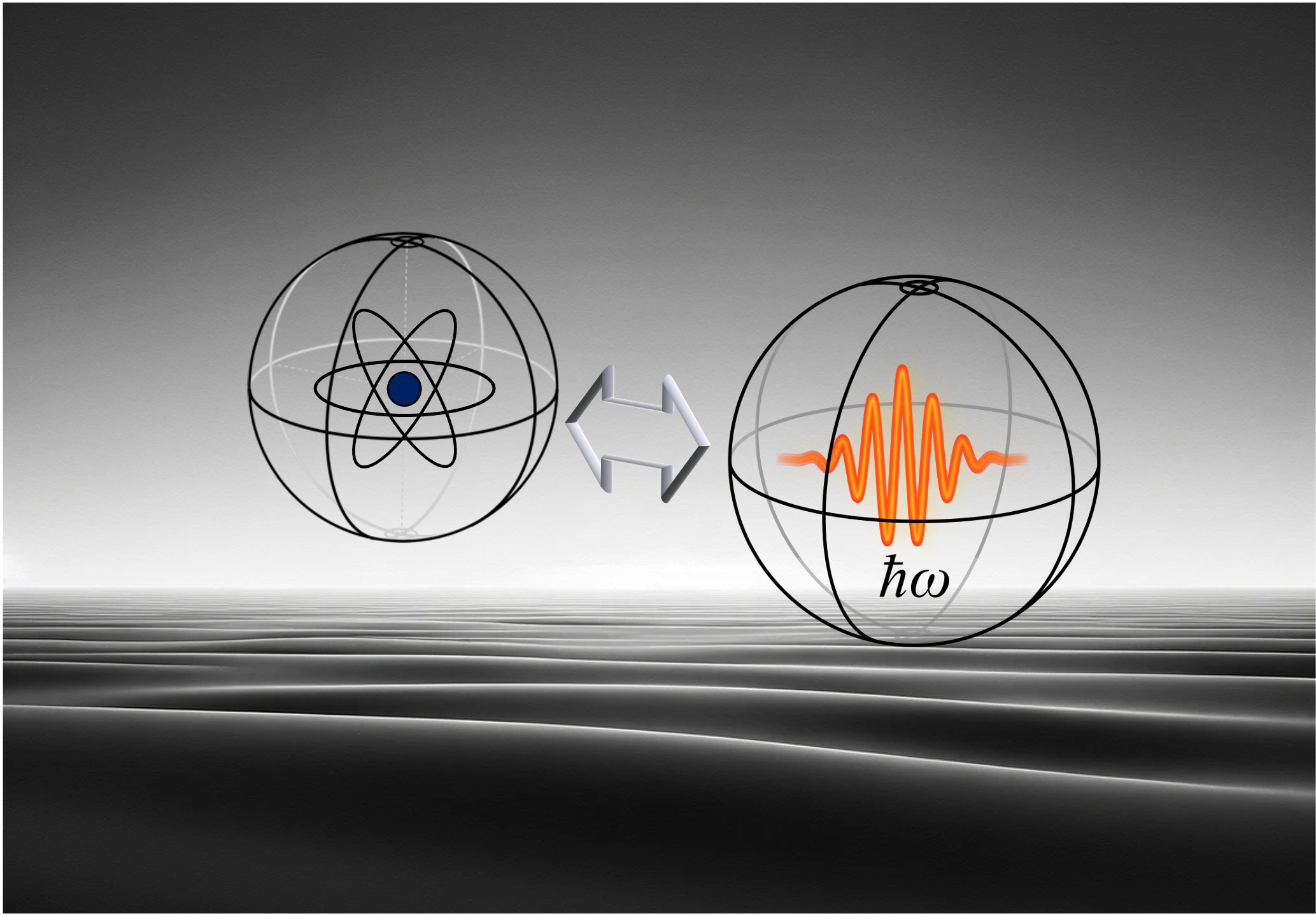
A passive photon-atom qubit SWAP gate
The first experimental demonstration of a qubit SWAP gate between two different types of qubits.

Extraction of a single photon from an optical pulse
Picking one photon from a laser pulse: a single-photon source for atom-mediated photonic quantum computation.
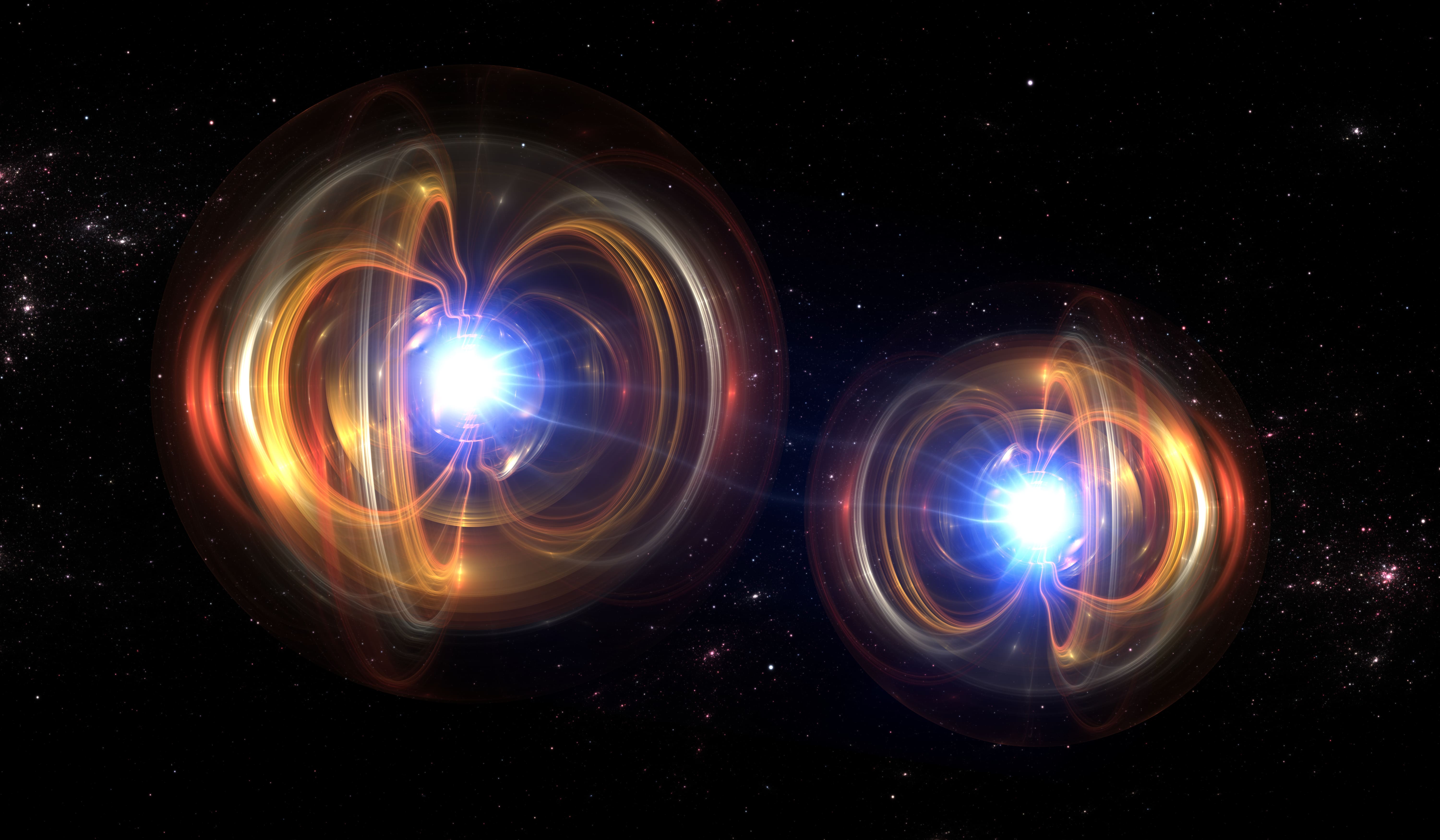
Analysis of deterministic swapping of photonic and atomic states
Single-Photon Raman Interaction (SPRINT) – the physics behind the qubit SWAP gate and single-photon extraction.
popular
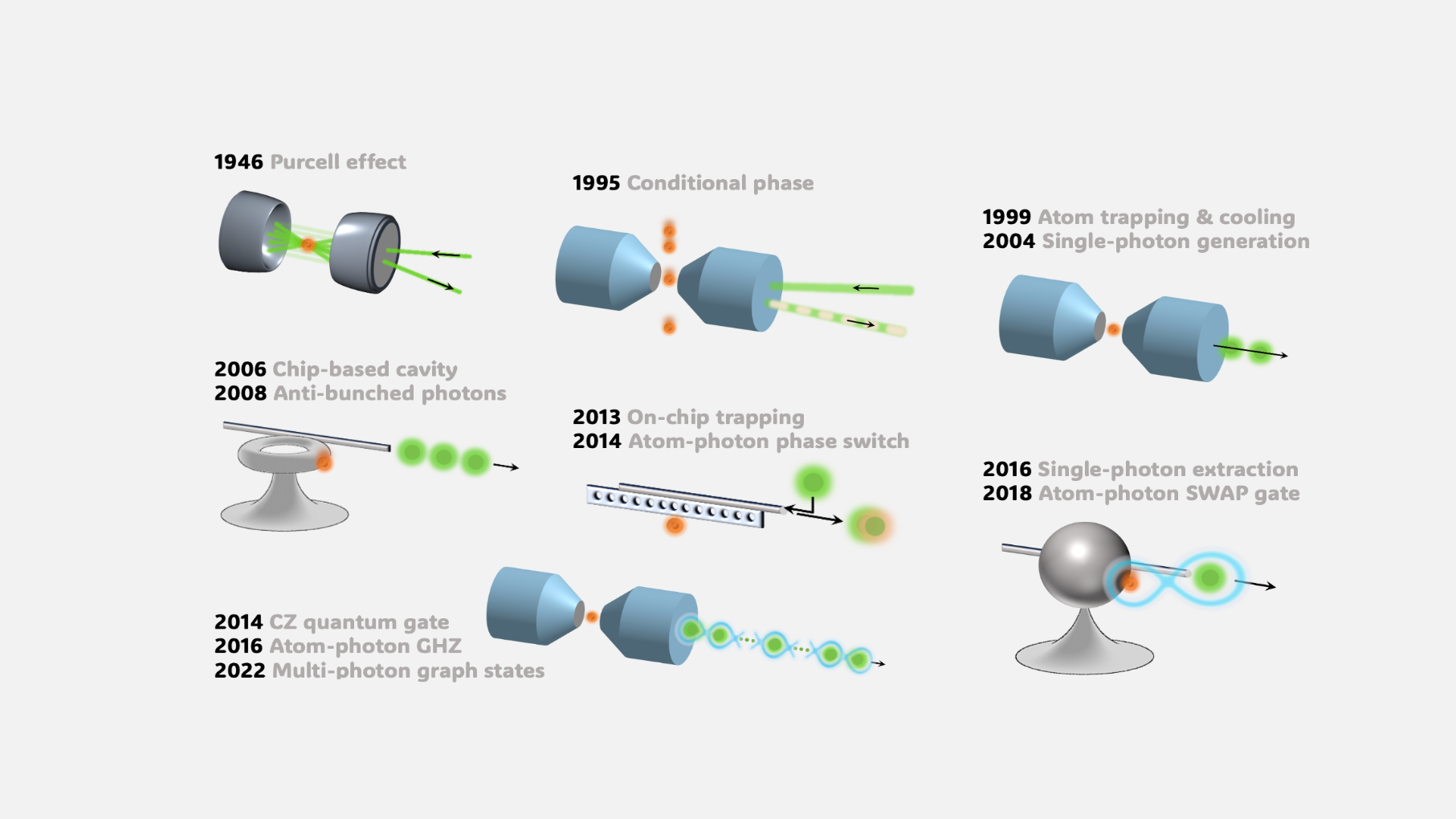
Controlling photons with atoms in a cavity: from bulk to integrated systems
This survey explores the evolution of cavity quantum electrodynamics (QED) concepts and technology over the years, transitioning from bulk optical structures and transient atoms to integrated fabrication-based resonators and stationary atoms.

SWAP - the most fundamental unit of communication in nature
Realizing a unitary exchange of qubits between light and matter carries both fundamental and practical significances.
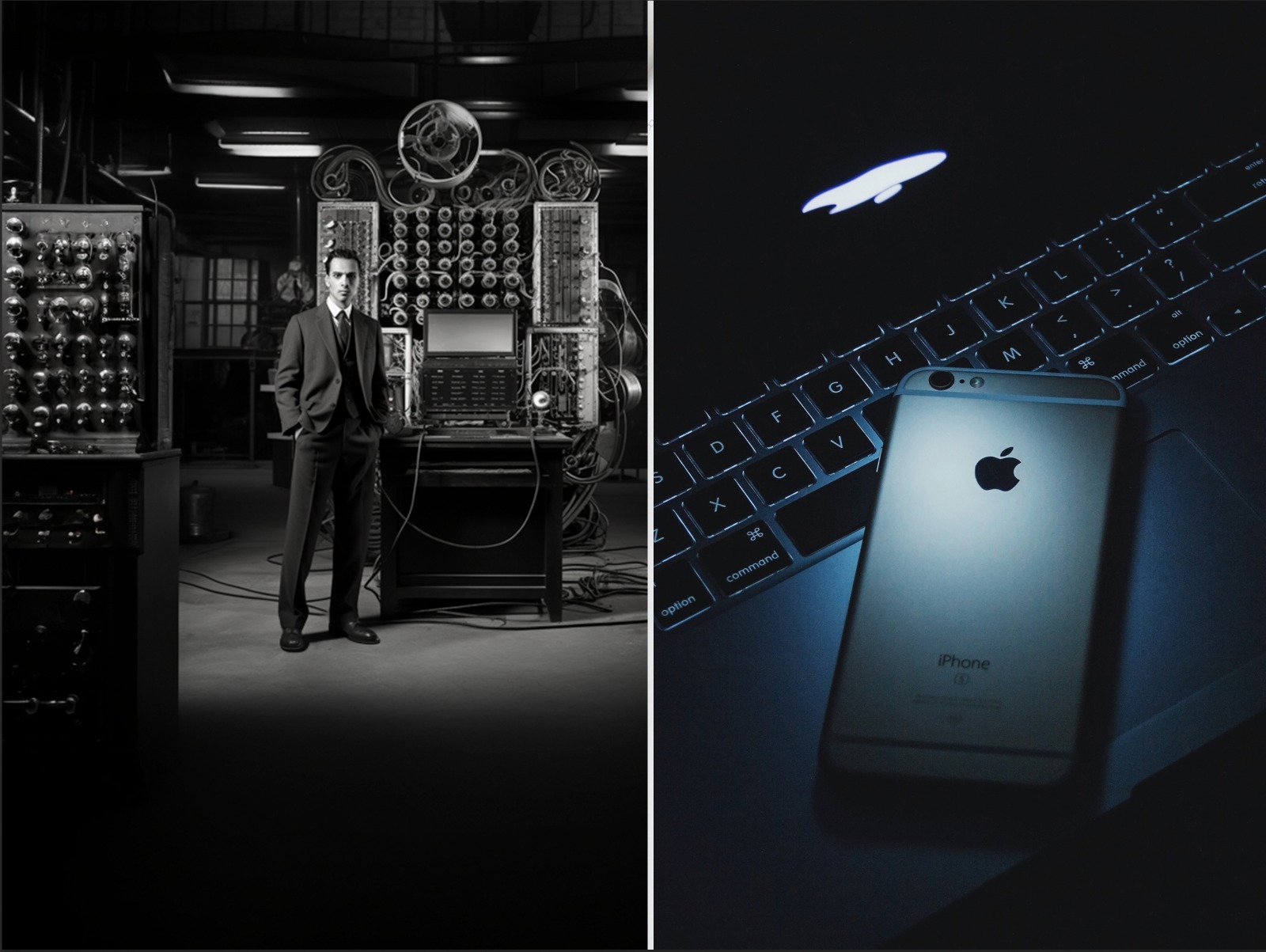
The meaning of practical & useful quantum computing
The real quantum revolution will begin when quantum computers become affordable and cost effective to scale. Quantum Source paves the way for practical, fault...
popular
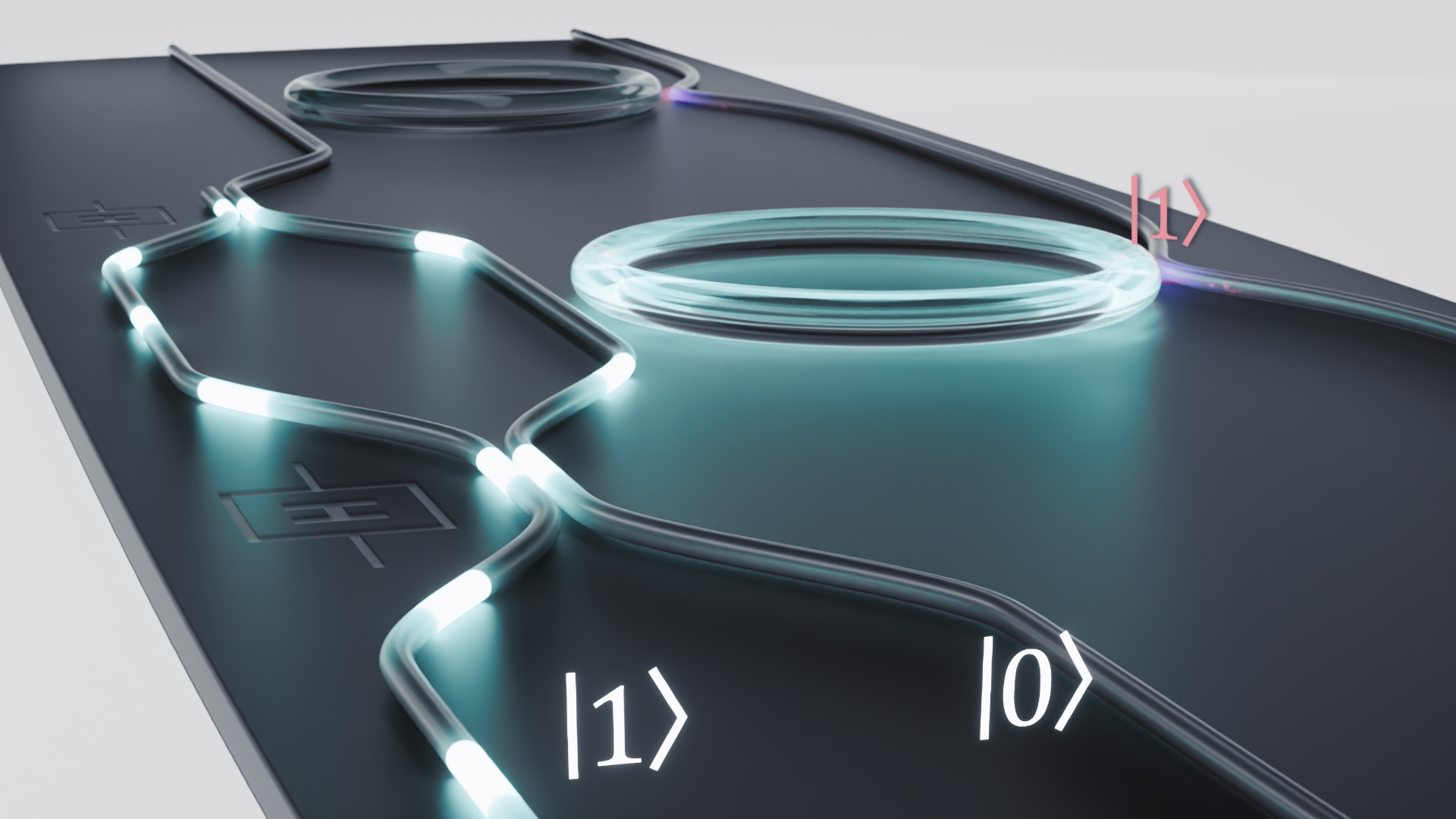
Quantum Source's practical path to million-qubit computations
By making resource state generation deterministic instead of probabilistic, Quantum Source overcomes existing quantum space and efficiency challenges.
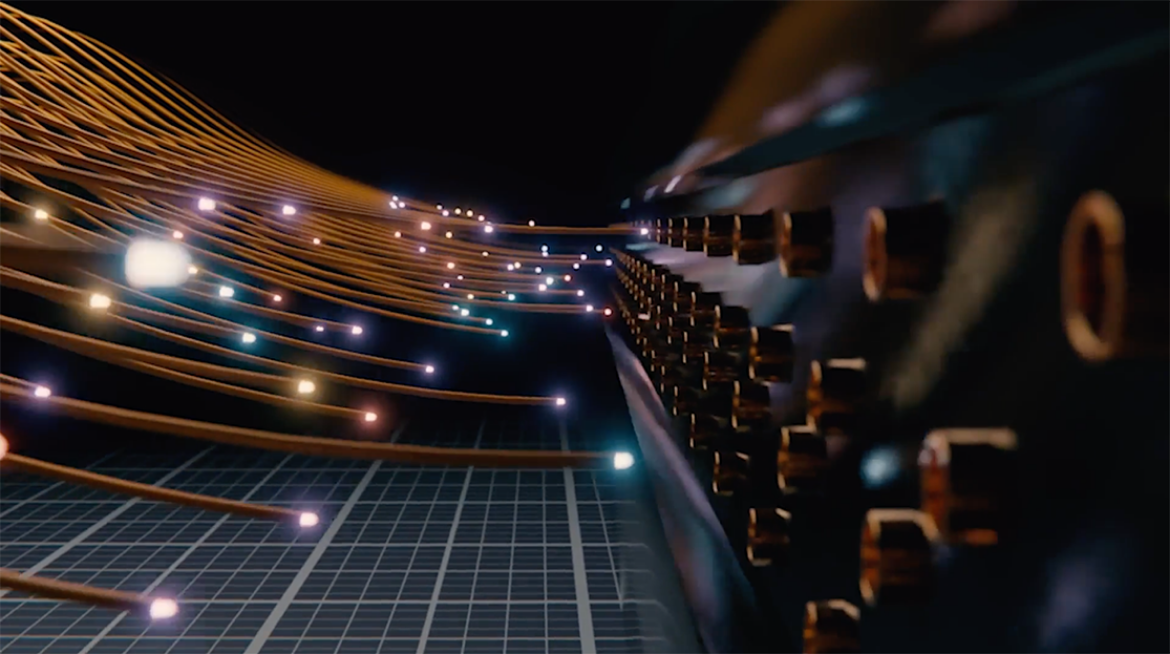
Photonics-based quantum computing
Photonic quantum computing offers the most promising route towards fault-tolerant quantum computers by providing a modular solution.
popular
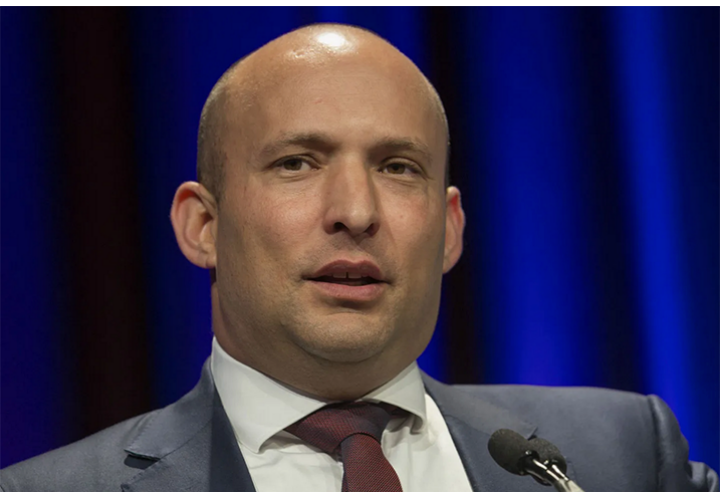
Former Israeli Prime Minister Naftali Bennett joins Quantum Source board of directors
Quantum Source Ltd., a pioneering quantum computing innovator, welcomes former Israeli Prime Minister Naftali Bennett to its board of directors.

Quantum Source raises $12M to propel continued development in photonic quantum computing
REHOVOT, Israel—(BUSINESS WIRE)—Quantum Source, an Israel-based company developing the technology needed to bring photonic quantum computers to market.

Quantum Source goes out of stealth to enable useful photonic quantum computers with millions of qubits
Three serial entrepreneurs and a leading Professor from the Weizmann Institute of Science join forces to establish Quantum Source and actualize a breakthrough.