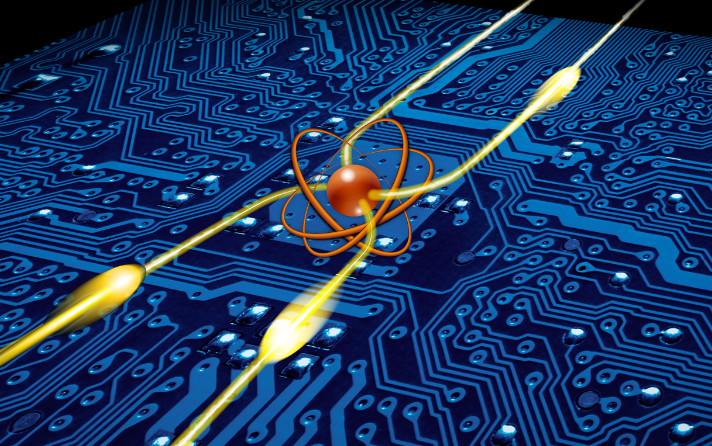
A photon controls a photon: a single atom router
The ability to control light by light, thereby achieving "all-optical" devices, has been a decades-long effort. One of the main goals of controlling light by light is to lower the switching energy, or in other words lower the number of photons required to toggle the state of the optical switch.
The experiment reported in Science in 2014 [1] is the first to achieve the most extreme case of an all-optical mechanism, namely controlling the state of the device solely by a single photon, without any additional control fields. In other words, this is the first realization of a single-photon photonic switch.
Such a sensitive response of a system to the smallest quanta of optical energy - a single photon - is a manifestation of what is called "single-photon nonlinearity", which can only be achieved by coupling light to a single quantum emitter, and specifically - a single atom.
The critical role of nonlinearity in computing
In order to understand the significance of controlling photons by photons, we should first consider the impact of a previous technological achievement: controlling electrons by electrons, more than 75 years ago.
In December 1947, three researchers from Bell Labs, John Bardeen, William Shockley and Walter Brattain, introduced the invention of the first transistor. Within less than a decade, the potential impact of this initially seeming academic demonstration became clear enough to earn the three inventors the 1956 Nobel prize in Physics.
The nonlinearity of this novel electronic component was initially harnessed mostly to perform amplification of electronic signals, including the well-known "transistor radios". However, as it turned out, the true impact of this invention was not the ability to use nonlinearity for amplification, but rather the ability to use it for logic operations - an ability that brought forth the dramatic rise of digital computers.
Similarly to electronics, in photonics, the first nonlinear devices are aimed at amplification purposes. From solid-state lasers to Erbium-doped fiber amplifiers, optical nonlinear devices form the backbone of modern communication today. The world is crisscrossed with over 10 billion kilometers of optical fibers, capable of delivering between terra-bytes to peta-bytes of information each. In fact, the vast majority of bits communicated by mankind every second nowadays pass at some point through an optical fiber.
Also with similarity to electronics, switching applications followed once the technological abilities became mature enough.
All-optical routing, in which optical signals control the flow of other optical signals, are used today to enable faster and more energy-efficient switching of communication packets without going to electronics and back, always aiming at higher nonlinear response that would accordingly lower the number of photons required in the control pulses.
However, the effort to follow the advancements performed in electronics and apply them in photonics is not trivial due to the drastic difference between the carriers of the information - the electron versus the photon. In contrast to electrons, photons practically do not interact with each other. Unfortunately, Star-Wars-like lightsabers are not realistic in normal conditions - one light beam cannot bend or even affect another light beam, unless there is some material medium involved.
Achieving nonlinearity at the single photon level
This brings us to the challenge of achieving nonlinearity all the way down to the single-photon level. The material system that needs to be involved needs to be a single emitter, like a single atom, for the response to be so nonlinear already at the level of a single photon. The problem is that a single atom is a very tiny "antenna" - it has a very small cross section that can interact with the incoming light. To make things worse, a single photon is a very tiny unit of energy. Usually, to control even single atoms scientists use laser pulses with many photons.
The key to overcoming this seemingly impossible challenge is to remember that a photon is indeed a tiny unit of energy, and the atom is a very tiny antenna, but that antenna responds to the electric field of the photon. Unlike the energy of the photon, which is a constant (E=hv), the electric field associated with every photon is not constant. If the single photon pulse is short, and it is focused to a tiny spot, the electric field has to be very large in order to keep the total amount of energy constant at hv. This is exactly the playground of cavity quantum electrodynamics (cavity-QED) - the quantum behavior of light and matter in highly confined cavities - waveguides and resonators.
Indeed, to enable this demonstration of a single-photon switch, the authors used all these mentioned 'ingredients': the photons were fiber-guided to a photonic chip with a micron-scale high-quality resonator. Such confinement increases the electric field associated with even a single photon to the level of high-power free space lasers. These intense electric fields allowed deterministic and fast interactions with single Rb atoms that were brought within ~100nm from the surface of this resonator. The result, as described in the paper, was a deterministic single-photon switch in which the first photon dictated whether the following photon will be reflected or transmitted.
Deterministic entanglement between photons will realize efficient fault-tolerant quantum computers
Like with electronic digital switching, this single-photon switching ability bears tremendous significance.
The same way in which the electronic switching brought forth the digital computer revolution, switching single photons by single photons can revolutionize the field of photonic quantum computation. The strong single-photon nonlinearity provided by such a system enables atom-mediated deterministic entanglement between photons, which is a crucial task, since clusters of entangled photons are the precious "fuel" of photonic quantum computation, and the generation of these clusters are by far the biggest challenge in realizing large-scale photonic quantum computers.
categories
popular
P-Atom-Mediated Deterministic Generation and Stitching of Photonic Graph States
How single atoms can solve the most demanding challenge in photonic quantum computation: deterministic generating of graph states
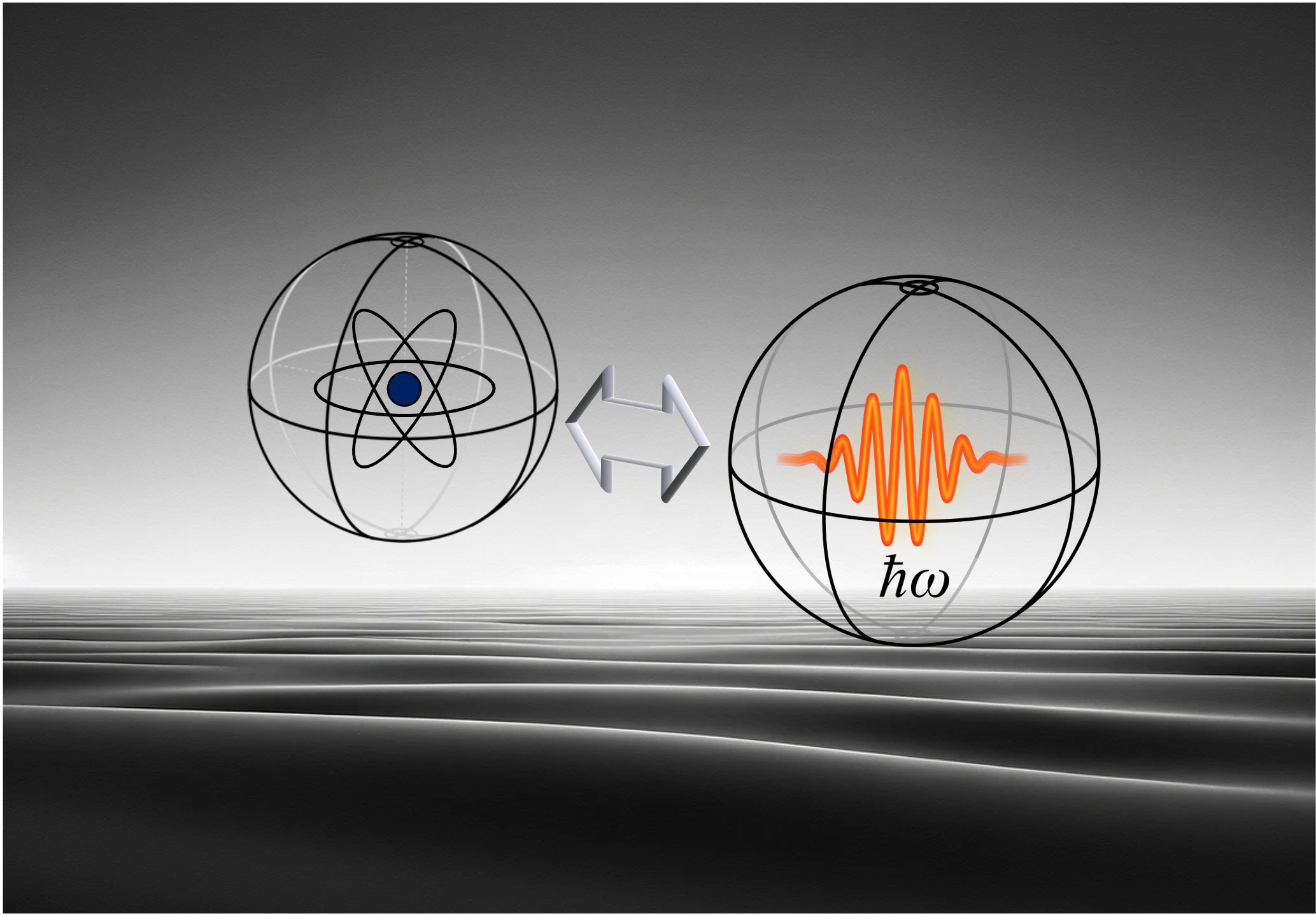
P-A passive photon-atom qubit SWAP gate
The first experimental demonstration of a qubit SWAP gate between two different types of qubits.
Similar
popular

V-Photonics-based quantum computing
Photonic quantum computing offers the most promising route towards fault-tolerant quantum computers by providing a modular solution.
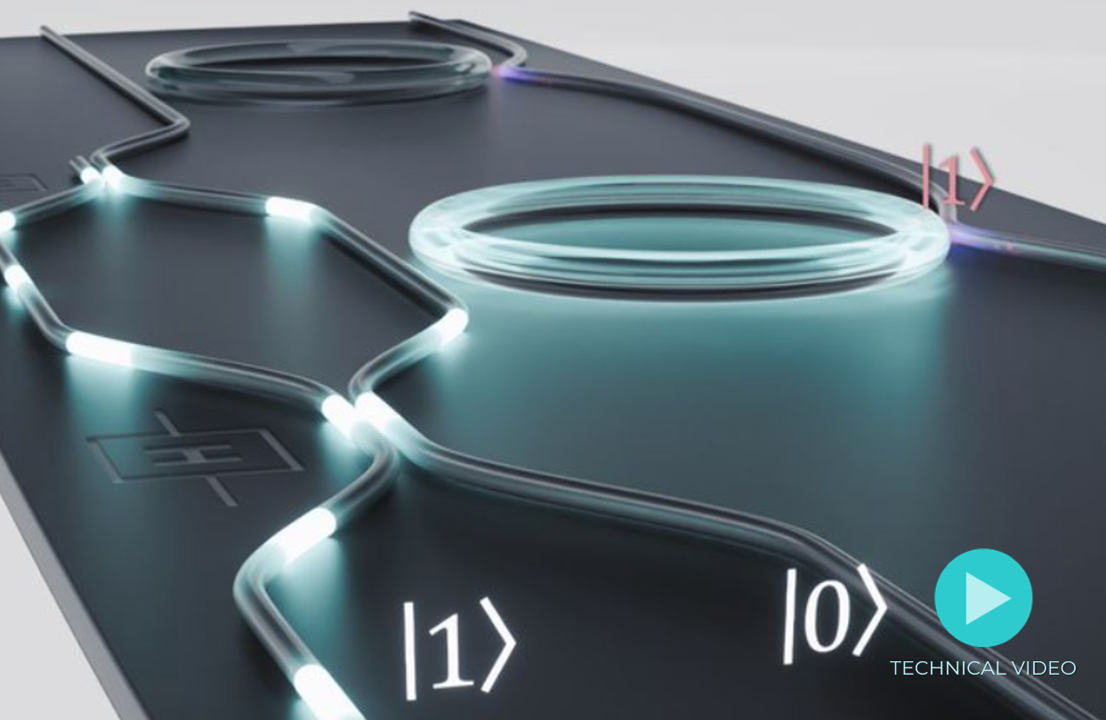
V-Quantum Source's practical path to million-qubit computations
By making resource state generation deterministic instead of probabilistic, Quantum Source overcomes existing quantum space and efficiency challenges.

V-Making Photonic Quantum Computation Scalable Using Single Atoms
At Q2B Tokyo 2025, our CEO Oded Melamed presented “Making Photonic Quantum Computation Scalable Using Single Atoms,” explaining how our photonic-based approach addresses key challenges to enable scalable quantum computing.
popular

N-Exclusive: Prof. Barak Dayan on Quantum Source’s Photon-Atom Breakthrough in Quantum Computing
In this TQI exclusive interview, Prof. Barak Dayan, Chief Scientist at Quantum Source, shares how their photon-atom technology is tackling some of the toughest challenges in quantum computing.

N-Quantum Source Raises $50 Million Series A Funding to Make Scalable, Useful Quantum Computing A Reality
Quantum Source, an Israel-based company developing, technology for powerful, cost-effective, practical photonic quantum computers, announced today it has raised a $50M Series A investment round led by Eclipse, with participation from Standard Investments, Level VC, Canon Equity, as well as existing investors Pitango First, Grove Ventures, 10D, and Dell Technologies Capital. This brings the company’s total funding raised to over $77M. The capital will be used to enhance engineering capabilities and accelerate the commercialization of quantum solutions.